Water Absorption Capacity of Flour
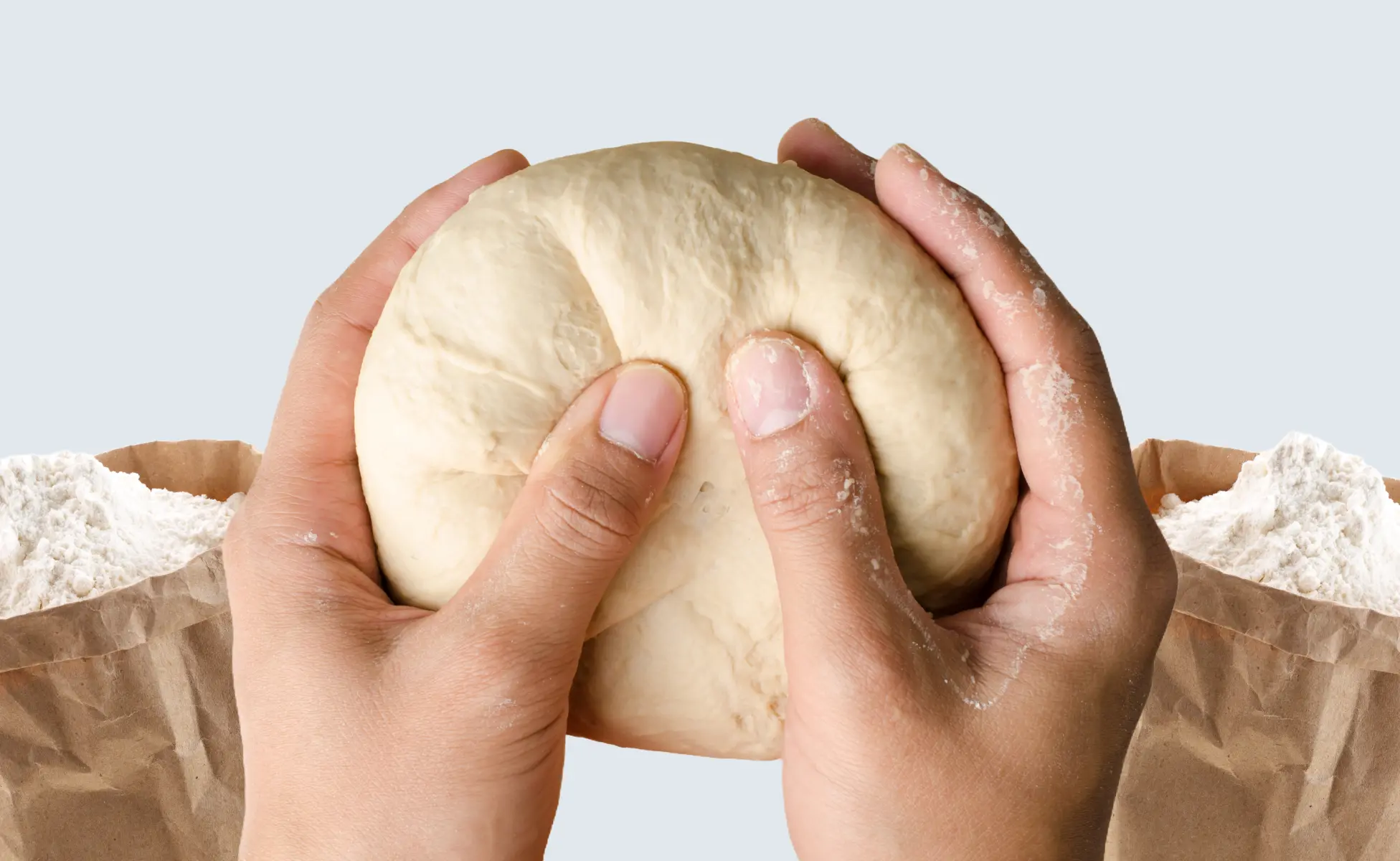
Why is Water Absorption Capacity Important?
It is an experience many of us have had when preparing a recipe using flour (bread, cake, pie, etc.). If you add too much water, the dough sticks to your hands and quickly make a mess. If you don't add enough water, the dough is easier to manipulate but is not stretchy enough. If this happens, our magnificent brioche stays, after fermentation, in the form of a small ball in the bottom of the baking pan. In short, we've all encountered problems with the water absorption capacity of flour.

At the individual baking level, one can add more flour to make a dough that is more firm or add more water to soften up dough that's too firm. However the consequences of an incorrect amount of water based on a flour's water absorption capacity in a manufacturing environment can create much more severe repercussions.
Everything else being equal, when secondary processing, there is a general economic interest in working with flour with a high water absorption capacity. The math is rather simple. If we can have the same finished product quality with flour that absorbs 65% water instead of 60%, we produce 5% more finished product by weight that only costs...the price of water!
The baker must avoid adding too much water; if the dough is too hydrated, it can become very sticky. And what is easily resolved in our kitchens will have great repercussions in automated factories and may even go so far as to halt production line, with significant financial impact.
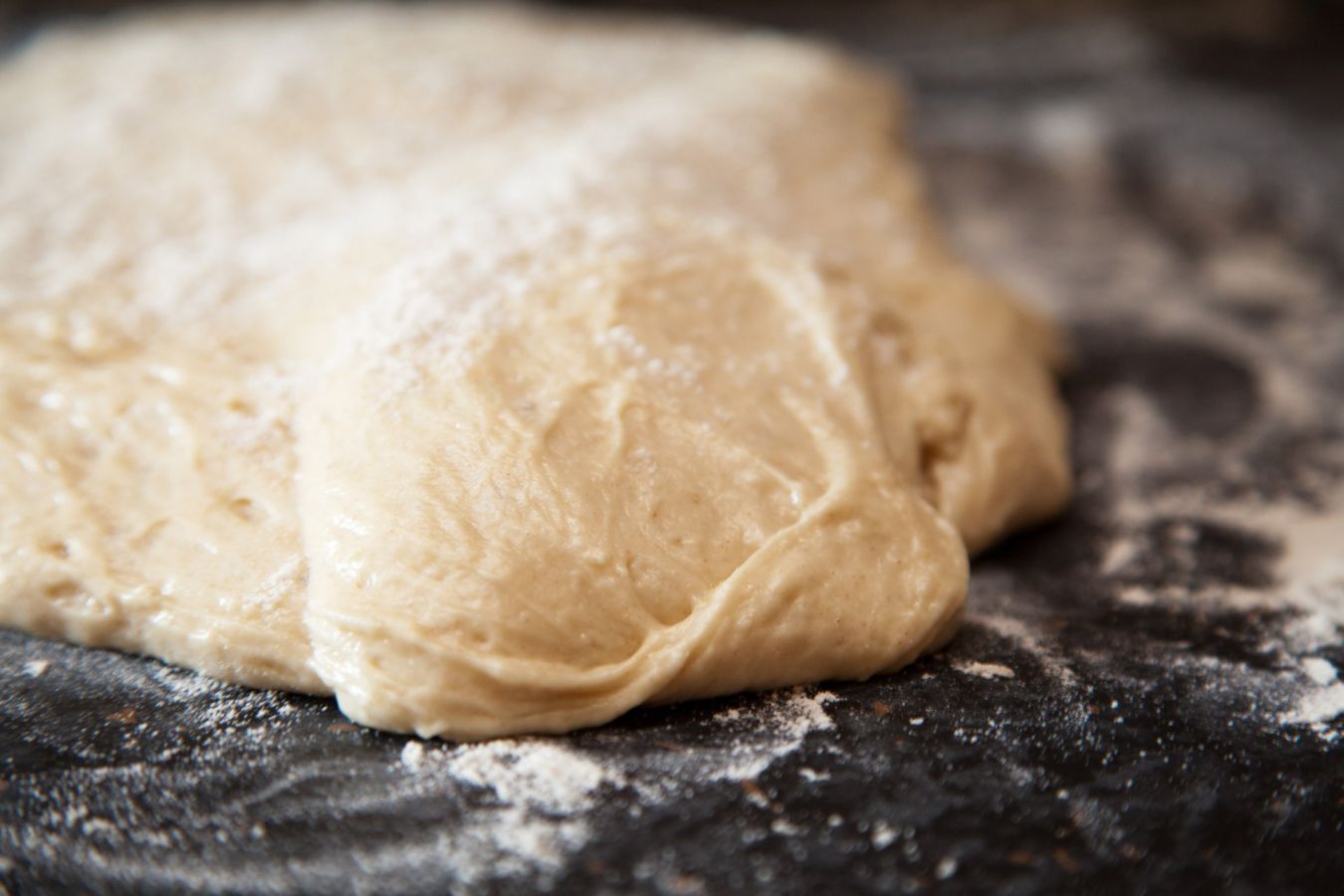
In practice, secondary processing needs flour with optimal and, above all, very consistent water absorption (i.e. the highest possible water absorption while allowing for the perfect functioning of production lines). Let us use the example of a production line running in an optimal manner with a fixed absorption rate of 60%. If the flour can only absorb 55%, the dough will be over-hydrated and will become sticky. If the flour can absorb 65%, the dough will be under-hydrated, which will impact the yield. In either case, the final quality of the product will probably not be optimal, and this type of situation requires the manufacturer to change their process settings with each new batch of flour...very inconvenient!
A good approach entails, quite simply, controlling each delivery of flour with a tool which allows for the measuring of the water absorption rate. Tools like the Mixolab 2 or the Consistograph are 100% suitable for this task.
Why do Different Flours Have Different Water Absorption Rates?
Wheat flour is primarily made up of starch, protein, water, lipids and mineral material. Apart from lipids, the four other components all have significant impact on water absorption.
The higher the moisture content of the flour (i.e., the more "water rich" it already is), the less room there is to add more. Conversely, the drier the flour, the more water it will absorb.
Mineral material, commonly referred to as "ash," is an indicator of the amount of the outer part of the grain (the bran) which ends up in the flour. The bran is very rich in compounds called pentosans. Although present in small quantities (approximately 1.5% of the flour), pentosans can absorb up to 15 times their weight in water. This also explains why whole wheat flour has a much higher water absorption capacity than "white" flour.
Protein/gluten constitutes between 7% and 17% (dry matter basis) of the flour. It has the capacity to absorb approximately two times its weight in water. The more protein/gluten, the more water absorption.
Starch makes up between 65% and 70% of the flour (dry matter basis). "Native" starch, undamaged, absorbs a relatively small amount of water (0.3 times its weight in water). In fact, it's more about the contact between the surface of the starch granule and the water (this is called "adsorption"). But during the process of transforming grain into flour, some of the starch granules end up damaged. Damaged starch granule's absorption potential increases from 0.3 to 3 times its weight in water. The more damaged starch, the more water absorption.
When water is added to the flour and it is mixed, there is competition between the different compounds to absorb the liquid. Damaged starch is the winner in this game (it's said to be very hygroscopic) and attracts water more quickly than protein. But damaged starch doesn't know how to retain the water and the water in turn is also released. The water then gets captured by the protein, which takes the opportunity to finalize its own hydration. However, if water continues to be released beyond the capacity retention of the protein, it will start to come out of the dough. This is when the dough becomes sticky.
In the end, a flour's water absorption capacity depends mainly on the work of the miller. He or she can raise or lower the water absorption by choosing and mixing different types of wheat, and preparing them differently for grinding, adjusting their grinding passage differently or by adding other ingredients, such as vital gluten.
The SDmatic 2 allows for the quick measurement of the quantity of damaged starch.
It remains clear that the water absorption rate cannot be viewed independently from the viscoelastic characteristics of the dough. It would be useless to produce flour with a very high absorption rate if it can't be used for bread making. We must ensure that the balance between water absorption rates and rheological properties is respected. This can be done with tools such as the Mixolab 2, the Alveograph (preferably with the adapted hydration protocol) or the RheoF4, for studying dough behavior during fermentation.
Related Blog Posts
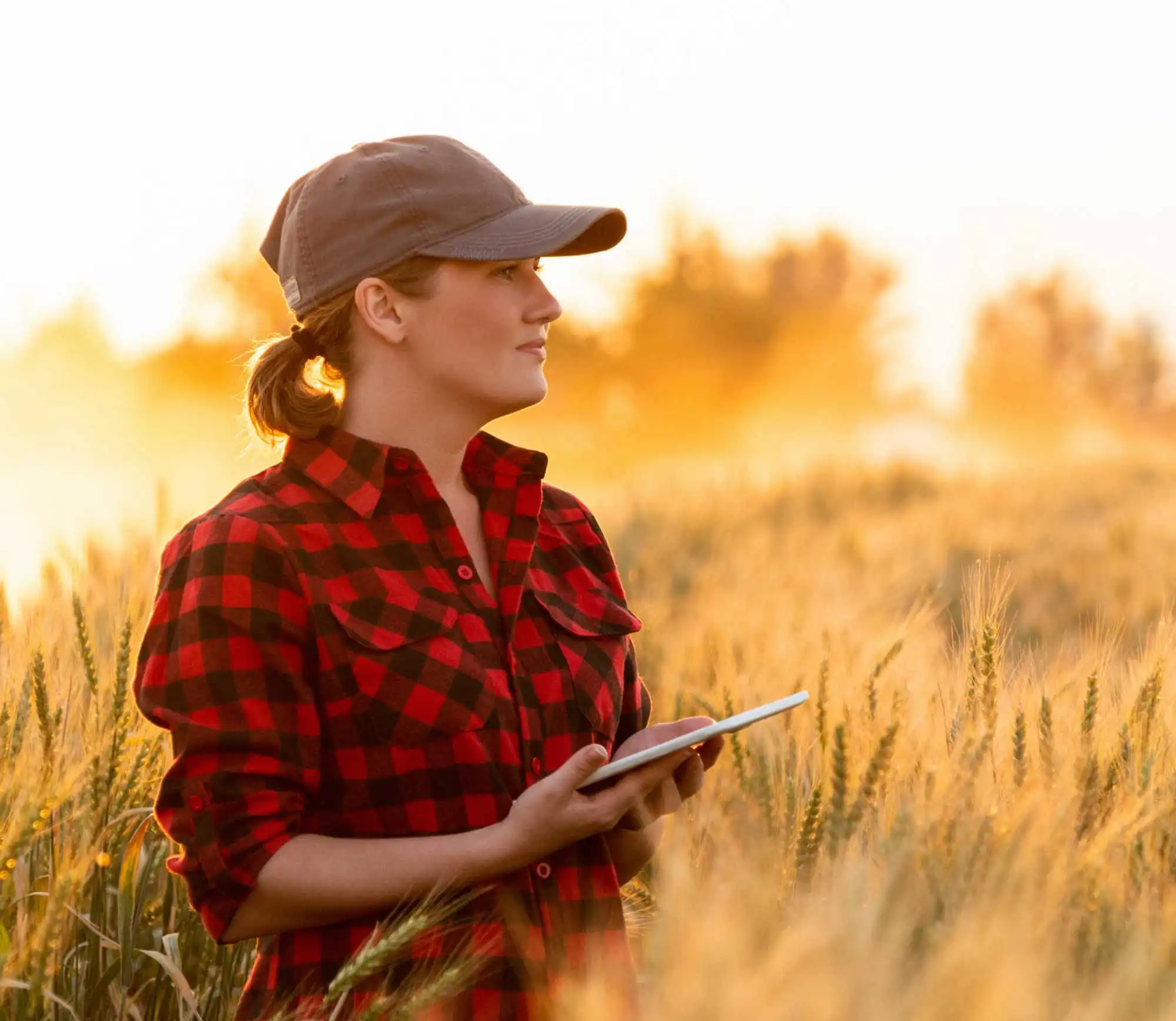
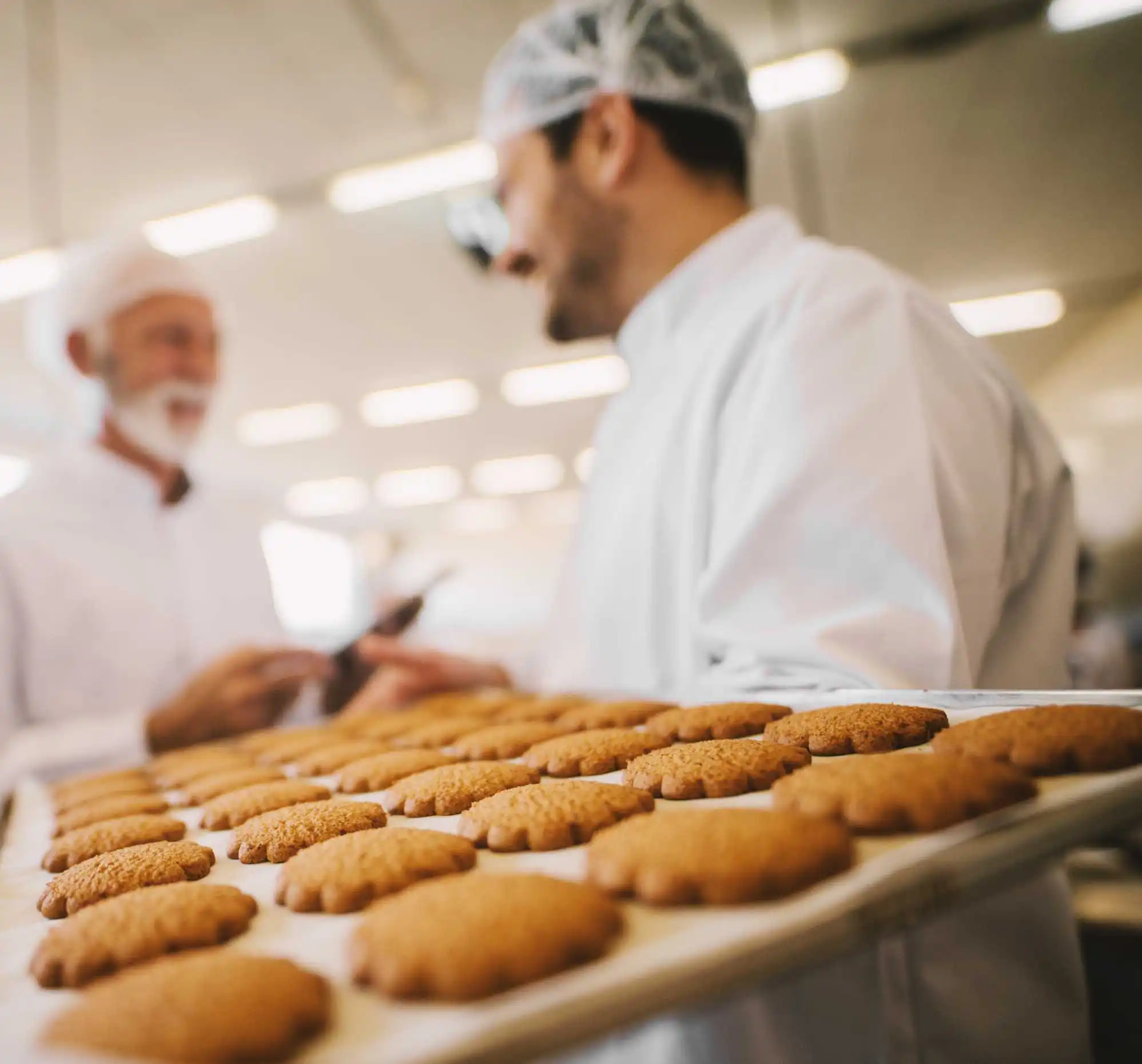

